A great challenge resides in using sunlight directly as the energy source for water splitting (i.e., without the intermediate production of electricity), thereby reproducing the direct light-to-chemical energetic transduction achieved by photosynthetic organisms. In such a process, light is used to extract electrons from water, which is oxidized to O2. Most organisms use these photogenerated electrons to reduce atmospheric carbon dioxide and produce carbohydrates, proteins or lipids as the main constituents of their biomass but some micro-organisms such as cyanobacteria or micro-algae are able, under very specific conditions, to photosynthesize hydrogen as well, in a fully sustainable way, called “light-driven water splitting”.
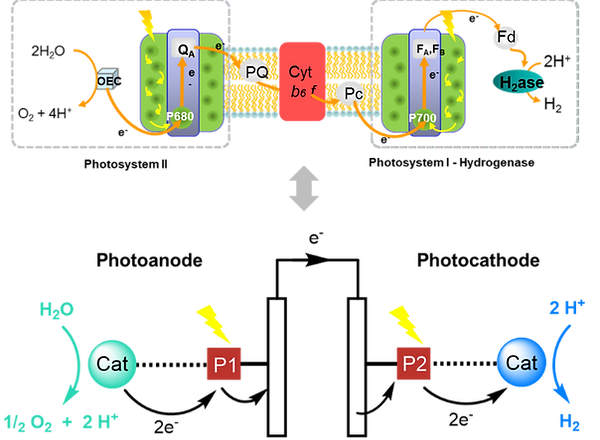
Taking inspiration from this naturally-occurring process, we are involved in the development of water splitting photoelectrochemical devices, combining bioinspired H2-evolving catalysts (developed in Axe I) with light-harvesting components, either solid-state systems (semi-conductors) or molecular photosensitizers (metallo-organic and fully organic dyes) grafted onto conducting materials.
We reported the first noble metal-free dye-sensitized H2-evolving photocathode based on the covalent assembly of the cobalt diimine dioxime catalyst with a push-pull organic dye. The resulting dyad was anchored onto mesoporous NiO films and the resulting dye-sensitized electrode produced H2 at pH 5.5 under continous visible light irradiation. We are currently involved in the rational optimization of the performances of these H2-evolving photocathodes built by a molecular strategy. Moreover, in collaboration with the groups of Pr. Ishitani (Tokyo Institute of Technology, Japan) and Pr. R. Abe ( Kyushu Univ, Japan), a molecular Z-scheme photoelectrochemical cell for water splitting combining a dye-sensitized photocathode with a low band-gap semiconductor photoanode was built.

From a fundamental point of view, these devices reproduce the key steps of photosynthesis that are light-harvesting, photoinduced spatial charge separation and multielectronic redox catalysis. Nevertheless, their efficiency is currently limited by an intrinsic property of the system: photoinduced electron transfers are innately monoelectronic whereas multielectronic catalysis is targeted. Nature overcomes this problem by means of an intermediate step of charge photoaccumulation which allows to temporarily store the reducing equivalents (or oxidants) involved in the reactions of interest. Integrating specific electron accumulation sites into artificial photosynthetic devices for solar fuel production is currently the subject of another project of the group. The reversible storage of two electrons coupled to two protons was observed on original ruthenium photosensitizers possessing pi-extended aromatic ligands, reproducing the mechanism at work with the plastoquinone electron relays in the electron transport chain of the photosynthetic apparatus.

Alternatively, the properties of inorganic semi-conductors can be exploited to produce efficient photocathodes. As a proof of concept, we demonstrated that the grafting of molecular catalysts onto TiO2 protected p-type silicon allows to fabricate quite stable photoelectrodes for H2 evolution with photocurrent densities reaching 1 mA/cm2 at 0 V vs RHE. The onset photopotentail of these hybrid photoelectrode is however too low and, we are searching for novel Earth-abundant semi-conductors thar could allow to overcome this limitation.
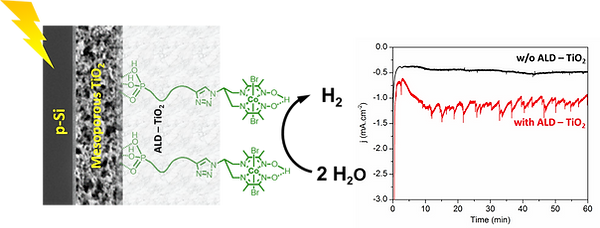
We also recently tackled the photoelectrochemical valorization of CO2. The true challenge is the capture of atmospheric CO2 and its conversion into a liquid fuel. This will requires breakthroughs in the design of suitable (photo)electrocatalytic materials. Our ambition is to develop novel (photo)electrode materials for selective CO2 reduction that would pave the way towards a sustainable solar-driven production of fuels and commodity chemicals.